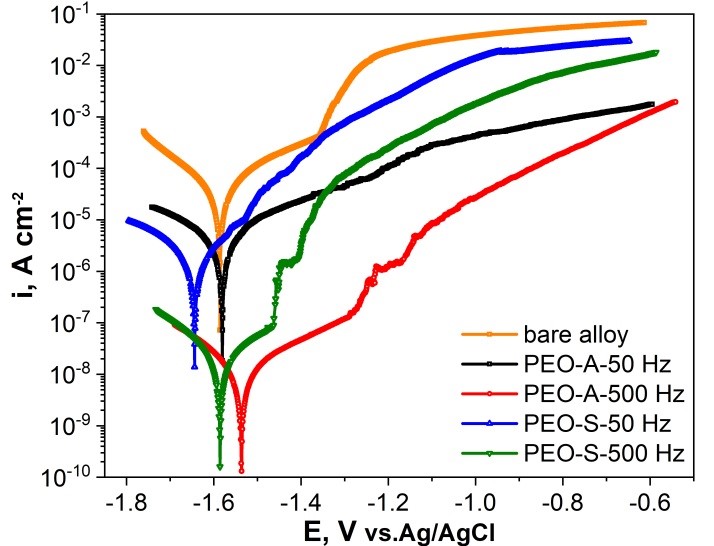
Improvement of protective oxide layers formed by high-frequency plasma electrolytic oxidation on Mg-RE alloy with LPSO-phase
Abstract
Keywords
Full Text:
PDFReferences
Song J, She J, Chen D, Pan F. Latest research advances on magnesium and magnesium alloys worldwide. J Magnes Alloy. 2020;8:1–41. doi:10.1016/j.jma.2020.02.003
Yang C, Cai H, Cui S, Huang J, Zhu J, Wu ZZ, Ma Z, Fu RKY, Sheng L, Tian X, Chu PK, Wu ZZ. A zinc-doped coating prepared on the magnesium alloy by plasma electrolytic oxidation for corrosion protection. Surf Coatings Technol. 2022;433:128148. doi:10.1016/j.surfcoat.2022.128148
Liu X, Liu L, Dong S, Chen X-B, Dong J. Towards dense corrosion-resistant plasma electrolytic oxidation coating on Mg-Gd-Y-Zr alloy by using ultra-high frequency pulse current. Surf Coatings Technol. 2022;447:128881. doi:10.1016/j.surfcoat.2022.128881
Wang SD, Xu DK, Han EH, Dong C. Stress corrosion cracking susceptibility of a high strength Mg–7%Gd–5%Y–1%Nd–0.5%Zr alloy. J Magnes Alloy. 2014;2:335–341. doi:10.1016/J.JMA.2014.11.004
Li CQ, Xu DK, Zeng ZR, Wang BJ, Sheng LY, Chen XB., Han EH. Effect of volume fraction of LPSO phases on corrosion and mechanical properties of Mg–Zn–Y alloys. Mater Des. 2017;121:430–441. doi:10.1016/j.matdes.2017.02.078
Wang SD, Xu DK, Wang BJ, Sheng LY, Qiao YX, Han EH, Dong C. Influence of phase dissolution and hydrogen absorption on the stress corrosion cracking behavior of Mg–7%Gd–5%Y–1%Nd–0.5%Zr alloy in 3.5 wt.% NaCl solution. Corros Sci. 2018;142:185–200. doi:10.1016/J.CORSCI.2018.07.019
Fattah-alhosseini A, Chaharmahali R, Babaei K, Nouri M, Keshavarz MK, Kaseem M. A review of effective strides in amelioration of the biocompatibility of PEO coatings on Mg alloys. J Magnes Alloy. 2022;10:2354–2383. doi:10.1016/j.jma.2022.09.002
Czerwinski F. Controlling the ignition and flammability of magnesium for aerospace applications. Corros Sci. 2014;86:1–16. doi:10.1016/J.CORSCI.2014.04.047
Xu D, Han EH, Xu Y. Effect of long-period stacking ordered phase on microstructure, mechanical property and corrosion resistance of Mg alloys: A review. Prog Nat Sci Mater Int. 2016;26:117–128. doi:10.1016/J.PNSC.2016.03.006
Qian Y, Zhao Y, Dong X, Yu W, Feng J, Yu H. Microstructure, Mechanical Properties and Fire Resistance of High Strength Mg-Gd-Y-Zr Alloys. Metals. 2022;12(9):1456. doi:10.3390/MET12091456
Wu J, Wu L, Yao W, Chen Y, Chen Y, Yuan Y, Wang J, Atrens A, Pan F. Effect of electrolyte systems on plasma electrolytic oxidation coatings characteristics on LPSO Mg-Gd-Y-Zn alloy. Surf Coatings Technol. 2023;454:129192. doi:10.1016/J.SURFCOAT.2022.129192
Wang G, Mao P, Wang Z, Zhou L, Wang F, Liu Z. High strain rates deformation behavior of an as-extruded Mg–2.5Zn–4Y magnesium alloy containing LPSO phase at high temperatures. J Mater Res. Technol. 2022;21:40–53. doi:10.1016/J.JMRT.2022.08.131
Volkova EF, Antipov VV, Zavodov AV. A Study of the Fine Structure and Phase Composition of Magnesium Alloy VMD16 in Cast and Homogenized Conditions. Met Sci Heat Treat. 2019;61:143–148. doi:10.1007/S11041-019-00390-6
Dai J, Dong Q, Nie Y, Jia Y, Chu C, Zhang X. Insight into the role of Y addition in the microstructures, mechanical and corrosion properties of as-cast Mg-Gd-Y-Zn-Ca-Zr alloys. Mater Des. 2022;221:110980. doi:10.1016/J.MATDES.2022.110980
Dang C, Wang J, Wang J, Yu D, Zheng W, Xu C, Lu R. Effect of lamellar LPSO phase on mechanical properties and damping capacity in cast magnesium alloys. J Mater Res Technol. 2023;22:2589–2599. doi:10.1016/J.JMRT.2022.12.087
Wu L, Li Y, Cheng Y, Linghu F, Jiang F, Chen G, Teng J, Fu D, Zhang H. Microstructure evolution and corrosion resistance improvement of Mg–Gd–Y–Zn–Zr alloys via surface hydrogen treatment. Corros Sci. 2021;191:109746. doi:10.1016/J.CORSCI.2021.109746
Liu Y, Wen J, Li H, He J. Effects of extrusion parameters on the microstructure, corrosion resistance, and mechanical properties of biodegradable Mg–Zn–Gd–Y–Zr alloy. J Alloys Compd. 2022;891:161964. doi:10.1016/J.JALLCOM.2021.161964
Zhang W, Zhao MC, Wang Z, Tan L, Qi Y, Yin DF, et al. Enhanced initial biodegradation resistance of the biomedical Mg-Cu alloy by surface nanomodification. J Magnes Alloy. 2022. doi:10.1016/J.JMA.2021.12.013
Zhang J, Xu J, Cheng W, Chen C, Kang J. Corrosion Behavior of Mg-Zn-Y Alloy with Long-period Stacking Ordered Structures. J Mater Sci Technol. 2012;28:1157–1162. doi:10.1016/S1005-0302(12)60186-8
Mohedano M, Pérez P, Matykina E, Pillado B, Garcés G, Arrabal R. PEO coating with Ce-sealing for corrosion protection of LPSO Mg–Y–Zn alloy. Surf Coatings Technol. 2020;383:125253. doi:10.1016/j.surfcoat.2019.125253
Kaseem M, Fatimah S, Nashrah N, Ko YG. Recent progress in surface modification of metals coated by plasma electrolytic oxidation: Principle, structure, and performance. Prog Mater Sci. 2020;117:100735. doi:10.1016/j.pmatsci.2020.100735
Polunin AV, Cheretaeva AO, Borgardt ED, Shafeev MR, Katsman AV, Krishtal MM. Influence of nanoparticle additions to the electrolyte on the structure, composition and corrosion resistance of oxide layers formed by PEO on cast Mg alloy. J Phys Conf Ser. 2020;1713:12036. doi:10.1088/1742-6596/1713/1/012036
Jangde A, Kumar S, Blawert C. Role of glycerine on formation & corrosion characteristic of PEO layer formed over Mg alloy in a high-concentrated mixed silicate-phosphate-based electrolyte. Surf Coatings Technol. 2022;450:128971. doi:10.1016/J.SURFCOAT.2022.128971
Yasui T, Hayashi K, Fukumoto M. Behaviors of Micro-Arcs, Bubbles, and Coating Growth during Plasma Electrolytic Oxidation of Titanium Alloy. Mater. 2023;16:360. doi:10.3390/MA16010360
Wu T, Blawert C, Lu X, Serdechnova M, Zheludkevich ML. Difference in formation of plasma electrolytic oxidation coatings on MgLi alloy in comparison with pure Mg. J Magnes Alloy. 2021;9:1725–1740. doi:10.1016/J.JMA.2021.03.017
Dehnavi V, Luan BL, Shoesmith DW, Liu XY, Rohani S. Effect of duty cycle and applied current frequency on plasma electrolytic oxidation (PEO) coating growth behavior. Surf Coatings Technol. 2013;226:100–107. doi:10.1016/j.surfcoat.2013.03.041
Kaseem M, Hussain T, Baek SH, Ko YG. Formation of stable coral reef-like structures via self-assembly of functionalized polyvinyl alcohol for superior corrosion performance of AZ31 Mg alloy. Mater Des. 2020;193. doi:10.1016/j.matdes.2020.108823
Fu W, Yang H, Li T, Sun J, Guo S, Fang D, et al. Enhancing corrosion resistance of ZK60 magnesium alloys via Ca microalloying: The impact of nanoscale precipitates. J Magnes Alloy. 2022. doi:10.1016/J.JMA.2022.06.011
Mohedano M, Lopez E, Mingo B, Moon S, Matykina E, Arrabal R. Energy consumption, wear and corrosion of PEO coatings on preanodized Al alloy: The influence of current and frequency. J Mater Res Technol. 2022;21:2061–2075. doi:10.1016/j.jmrt.2022.10.049
Lv GH, Chen H, Gu WC, Li L,Niu EW, Zhang XH, Yang SZ. Effects of current frequency on the structural characteristics and corrosion property of ceramic coatings formed on magnesium alloy by PEO technology. J Mater Process Technol. 2008;208:9–13. doi:10.1016/J.JMATPROTEC.2007.12.125
Srinivasan PB, Liang J, Balajeee RG, Blawert C, Störmer M, Dietzel W. Effect of pulse frequency on the microstructure, phase composition and corrosion performance of a phosphate-based plasma electrolytic oxidation coated AM50 magnesium alloy. Appl Surf Sci. 2010;256:3928–3935. doi:10.1016/J.APSUSC.2010.01.052
Li J, Bian Y, Tu X, Li W, Song D. Influence of surface roughness of substrate on corrosion behavior of MAO coated ZM5 Mg alloy. J Electroanal Chem. 2022;910:116206. doi:10.1016/J.JELECHEM.2022.116206
Zhu M, Song Y, Dong K, Shan D, Han EH. Correlation between the transient variation in positive/negative pulse voltages and the growth of PEO coating on 7075 aluminum alloy. Electrochim Acta. 2022;411:140056. doi:10.1016/J.ELECTACTA.2022.140056
Zhang Z, Liu X, Wang Z, Le Q, Hu W, Bao L, et al. Effects of phase composition and content on the microstructures and mechanical properties of high strength Mg–Y–Zn–Zr alloys. Mater Des. 2015;88:915–923. doi:10.1016/J.MATDES.2015.09.087
Hakimizad A, Raeissi K, Santamaria M, Asghari M. Effects of pulse current mode on plasma electrolytic oxidation of 7075 Al in Na2WO4 containing solution: From unipolar to soft-sparking regime. Electrochim Acta. 2018;284:618–629. doi:10.1016/j.electacta.2018.07.200
Kaseem M, Kamil MP, Ko YG. Electrochemical response of MoO2-Al2O3 oxide films via plasma electrolytic oxidation. Surf Coatings Technol. 2017;322:163–173. doi:10.1016/J.SURFCOAT.2017.05.051
Duan H, Yan C, Wang F. Effect of electrolyte additives on performance of plasma electrolytic oxidation films formed on magnesium alloy AZ91D. Electrochim Acta. 2007;52:3785–3793. doi:10.1016/j.electacta.2006.10.066
Ghasemi A, Raja VS, Blawert C, Dietzel W, Kainer KU. The role of anions in the formation and corrosion resistance of the plasma electrolytic oxidation coatings. Surf Coatings Technol. 2010;204:1469–1478. doi:10.1016/j.surfcoat.2009.09.069
Gulec AE, Gencer Y, Tarakci M. The characterization of oxide based ceramic coating synthesized on Al-Si binary alloys by microarc oxidation. Surf Coatings Technol. 2015;269:100–107. doi:10.1016/j.surfcoat.2014.12.031
Li K, Li W, Zhang G, Zhu W, Zheng F, Zhang D, et al. Effects of Si phase refinement on the plasma electrolytic oxidation of eutectic Al-Si alloy. J Alloys Compd. 2019;790:650–656. doi:10.1016/J.JALLCOM.2019.03.217
Saheb N, Lamara S, Sahnoune F, Hassan SF. Kinetic analysis of the formation of magnesium aluminate spinel (MgAl2O4) from α-Al2O3 and MgO nanopowders. J Therm Anal Calorim. 2022;147:11549–11559. doi:10.1007/S10973-022-11344-1/TABLES/5
Mikhail SA, King PE. High-temperature thermal analysis study of the reaction between magnesium oxide and silica. J Therm Anal. 1993;40:79–84. doi:10.1007/BF02546557
Zehra T, Patil SA, Shrestha NK, Fattah-alhosseini A, Kaseem M. Anionic assisted incorporation of WO3 nanoparticles for enhanced electrochemical properties of AZ31 Mg alloy coated via plasma electrolytic oxidation. J Alloys Compd. 2022;916:165445. doi:10.1016/J.JALLCOM.2022.165445
Toulabifard A, Rahmati M, Raeissi K, Hakimizad A, Santamaria M. The Effect of Electrolytic Solution Composition on the Structure, Corrosion, and Wear Resistance of PEO Coatings on AZ31 Magnesium Alloy. Coatings. 2020;10:937. doi:10.3390/COATINGS10100937
Yerokhin AL, Nie X, Leyland A, Matthews A, Dowey SJ. Plasma electrolysis for surface engineering. Surf Coatings Technol. 1999;122:73–93. doi:10.1016/S0257-8972(99)00441-7
Nominé AV,Troughton SC, Nominé AV, Henrion G, Clyne TW. High speed video evidence for localised discharge cascades during plasma electrolytic oxidation. Surf Coatings Technol. 2015;269:125–130. doi:10.1016/j.surfcoat.2015.01.043
Dunleavy CS, Golosnoy IO, Curran JA, Clyne TW. Characterisation of discharge events during plasma electrolytic oxidation. Surf Coatings Technol. 2009;203:3410–3419. doi:10.1016/j.surfcoat.2009.05.004
Jovović J, Stojadinović S, Šišović NM, Konjević N. Spectroscopic study of plasma during electrolytic oxidation of magnesium- and aluminium-alloy. J Quant Spectrosc Radiat Transf. 2012;113:1928–1937. doi:10.1016/j.jqsrt.2012.06.008
Sundararajan G, Rama Krishna L. Mechanisms underlying the formation of thick alumina coatings through the MAO coating technology. Surf Coatings Technol. 2003;167:269–277. doi:10.1016/S0257-8972(02)00918-0
Troughton SC, Clyne TW. Cathodic discharges during high frequency plasma electrolytic oxidation. Surf Coatings Technol. 2018;352:591–599. doi:10.1016/j.surfcoat.2018.08.049
Hussein RO, Nie X, Northwood DO. An investigation of ceramic coating growth mechanisms in plasma electrolytic oxidation (PEO) processing. Electrochim Acta. 2013;112:111–119. doi:10.1016/J.ELECTACTA.2013.08.137
Pyachin SA, Burkov AA, Kaminskii OI, Zaikova ER. Melting of a Titanium Alloy Under the Action of Electrical Discharges of Different Duration. Russ Phys J. 2019;61:2236–2243. doi:10.1007/S11182-019-01661-8
Ren L, Grew ES, Xiong M, Ma Z. Wagnerite-Ma5bc, a new polytype of Mg2(PO4)(F,OH), from granulite-facies Paragneiss, Larsemann Hills, Prydz Bay, East Antarctica. Can Mineral. 2003;41:393–411. doi:10.2113/GSCANMIN.41.2.393
Duan H, Du K, Yan C., Wang F. Electrochemical corrosion behavior of composite coatings of sealed MAO film on magnesium alloy AZ91D. Electrochim Acta. 2006;51:2898–2908. doi:10.1016/j.electacta.2005.08.026
Hwang DY, Kim YM, Shin DH. Corrosion resistance of plasma-anodized AZ91 Mg alloy in the electrolyte with/without potassium fluoride. Mater Trans. 2009;50:671–678. doi:10.2320/MATERTRANS.MER2008345
Lujun Z, Hongzhan H, Qingmei M, Jiangbo L, Zhengxian L. The mechanism for tuning the corrosion resistance and pore density of plasma electrolytic oxidation (PEO) coatings on Mg alloy with fluoride addition. J Magnes Alloy. 2021. doi:10.1016/J.JMA.2021.10.007
Chen Q, Zheng Y, Dong S, Chen XB, Dong J. Effects of fluoride ions as electrolyte additives for a PEO/Ni-P composite coating onto Mg alloy AZ31B. Surf Coatings Technol. 2021;417:126883. doi:10.1016/j.surfcoat.2021.126883
DOI: https://doi.org/10.15826/chimtech.2023.10.2.12
Copyright (c) 2023 Alisa O. Cheretaeva, Pavel A. Glukhov, Marat R. Shafeev, Alyona G. Denisova, Eugeny D. Borgardt, Anton V. Polunin, Alexander V. Katsman, Mikhail M. Krishtal

This work is licensed under a Creative Commons Attribution 4.0 International License.
© Website Chimica Techno Acta, 2014–2024
ISSN 2411-1414 (Online)
This journal is licensed under a Creative Commons Attribution 4.0 International