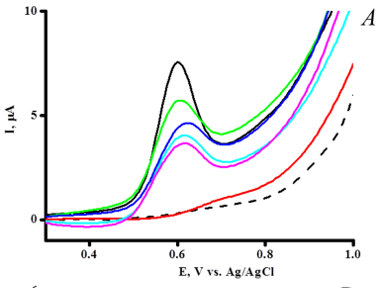
Electrochemical sensor for the detection of serine β-lactamase catalytic activity
Abstract
Keywords
Full Text:
PDFReferences
Bottari F, Blust R, De Wael K. Bio(inspired) strategies for the electro-sensing of β-lactam antibiotics. Curr Opin Electrochem. 2018;10:136–142. doi:10.1016/j.coelec.2018.05.015
Zdarska V, Kolar M, Mlynarcik P. Occurrence of beta-lactamases in bacteria. Infect Genet Evol. 2024;122:105610. doi:10.1016/j.meegid. 2024.105610
Kang SJ, Kim DH, Lee BJ. Metallo-β-lactamase inhibitors: A continuing challenge for combating antibiotic resistance. Biophys Chem. 2024;309:107228. doi:10.1016/j.bpc.2024.107228
Giguère S, Prescott JF. Antimicrobial therapy in veterinary medicine. In: Dowling P.M. editor. 5th ed. Wiley and Sons. Inc.; 2013. doi:10.1002/9781118675014
Zhuang Q, Guo H, Peng T, Ding E, Zhao H, Liu Q et al. Advances in the detection of β-lactamase: A review. Int J Biol Macromol. 2023;251:126159. doi:10.1016/j.ijbiomac.2023.126159
Mojica MF, Rossi MA, Vila AJ. The urgent need for metallo-β-lactamase inhibitors: an unattended global threat. Lancet Infect Dis. 2022;.22:e28–e34. doi:10.1016/S1473-3099(20)30868-9
Blair JMA, Webber MA, Baylay AJ, Ogbolu DO, Piddock LJV. Molecular mechanisms of antibiotic resistance. Nat. Rev Microbiol. 2015;13:42–51. doi:10.1038/nrmicro3380
Egorov АМ, Ulyashova ММ, Rubtsova MYu. Bacterial enzymes and antibiotic resistance. Acta Nat. 2018;10:33–48. doi:10.32607/20758251-2018-10-4-33-48
Bush K. Past and present perspectives on β-lactamases. Antimicrob Agents Chemother. 2018;62:1076–1118. doi:10.1128/AAC.01076-18
Egorov A, Ulyashova M, Rubtsova M.Impact of Key and Secondary Drug Resistance Mutations on Structure and Activity of β-Lactamases. In: Antibiotic Drug Resistance. Wiley. 2019;119–140. doi:10.1002/9781119282549.ch6
Babic M, Hujer AM, Bonomo RA. What ’s new in antibiotic resistance? Focus on beta-lactamases. Drug Resist Updat. 2006;9:142–156. doi:10.1016/j.drup.2006.05.005
Castanheira M, Simner PJ, Bradford PA. Extended-spectrum β-lactamases: an update on their characteristics, epidemiology and detection. JAC-Antimicrob Resist. 2021;3:dlab092. doi:10.1093/jacamr/dlab092
Qais FA, Ahmad I. In vitro interaction of cefotaxime withcalf thymus DNA: Insights from spectroscopic, calorimetric and molecular modelling studies. J Pharm Biomed Anal. 2018;149:193–205. doi:10.1016/j.jpba.2017.10.016
Fursova N, Pryamchuk S, Kruglov A. The novel CTX-M-116 β-lactamase gene discovered in Proteus mirabilis is composed of parts of the CTX-M-22 and CTX-M-23 genes. Antimicrob Agents Chemother. 2013;57:1552–1555. doi:10.1128/AAC.01471-12
Liu G, Li W, Li S, Xu J, Wang X, Xu H, et al. Culture-free detection of β-lactamase-Producing bacteria in urinary tract infections using a paper sensor. Biosens Bioelectron. 2024;257:116300. doi:10.1016/j.bios.2024.116300
Grigorenko VG, Andreeva IP, Rubtsova MY, Deygen I M, Antipin RL, Majouga AG, et al. Novel Non-β-Lactam Inhibitor of β-Lactamase TEM-171 Based on Acylated Phenoxyaniline. Biochimie. 2017; 132: 45–53. doi:10.1016/j.biochi.2016.10.011
Dos Santos M, Wrobel E, dos Santos V, Quinaia SP, Fujiwara ST, Garcia JR, et al. Development of an electrochemical sensor based on LbL films of Pt nanoparticles and humic acid. J Electrochem Soc. 2016;163:499–506. doi:10.1149/2.1001609jes
Wang J. Analytical Electrochemistry, 3rd ed.; JohnWiley & Sons, Inc.: Hoboken, NJ, USA, 2006; p. 32. P. 202. doi:10.1002/0471790303
Shumyantseva V, Bulko T, Pronina V, Kanashenko S, Pokrovskaya M, Aleksandrova S, et al. Electroenzymatic Model System for the Determination of Catalytic Activity of Erwinia carotovora L-Asparaginase. Processes. 2022;10:1313. doi:10.3390/pr10071313
Shumyantseva VV, Kuzikov AV, Masamrekh RA, Bulko TV, Archakov AI. From electrochemistry to enzyme kinetics of cytochrome P450. Biosens Bioelectron. 2018;15:192–204. doi:10.1016/j.bios.2018.08.040
Koroleva P., Bulko TV, Agafonova LE, Shumyantseva VV. Catalytic and Electrocatalytic Mechanisms of Cytochromes P450 in the Development of Biosensors and Bioreactors. Biochemistry(Mosc). 2023;88:1645-1657. doi:10.1134/S0006297923100176
Mi L, Wang Z, Yang W, Huang C, Zhou B, Hu Y, et al. Cytochromes P450 in biosensing and biosynthesis applications: Recent progress and future perspectives. TrAC. 2023;158:116791. doi:10.1016/j.trac.2022.116791
Filippova TA, Masamrekh RA, Shumyantseva VV, Latsis IA, Farafonova TE, Ilina IY, et al. Electrochemical biosensor for trypsin activity assay based on cleavage of immobilized tyrosine-containing peptide. Talanta. 2023;257:124341. doi:10.1016/j.talanta.2023.124341
Sfragano PS, Moro G, Polo F, Palchetti I. The role of peptides in the design of electrochemical biosensors for clinical diagnostics. Biosensors (Basel). 2021;11:246. doi:10.3390/bios11080246
Rodriguez-Rios M, Megia-Fernandez A, Norman DJ, Bradley M. Peptide probes for proteases – Innovations and applications for monitoring proteolytic activity. Chem Soc Rev. 2022;51:2081–2120. doi:10.1039/d1cs00798j
Shumyantseva V, Agafonova L, Bulko T, Kuzikov A, Masamrekh R, Yuan J, et al.Electroanalysis of Biomolecules: Rational Selection of Sensor Construction. Biochem (Mosc). 2021;86:S140–S151. doi:10.1134/S0006297921140108
Sumitha MS, Xavier TS. Recent advances in electrochemical biosensors – A brief review. Hybrid Adv. 2023;2:100023. doi:10.1016/j.hybadv.2023.100023
García-Miranda Ferrari A, Rowley-Neale SJ, Banks CE. Screen-printed electrodes: Transitioning the laboratory in-to-the field. Talanta Open. 2021;3:100032. doi:10.1016/j.talo.2021.100032
Paimard G, Ghasali E, Baeza M. Screen-printed electrodes: Fabrication, modification, and biosensing applications. Chemosensors. 2023;11:113. doi:10.3390/chemosensors11020113
Carrara S, Baj-Rossi C, Boero C, De Micheli G. Do Carbon Nanotubes contribute to Electrochemical Biosensing? Electrochim Acta. 2014;128:102–112. doi:10.1016/j.electacta.2013.12.123
Alim S, Vejayan J, Yusoff MM, Kafi AKM. Recent uses of carbon nanotubes & gold nanoparticles in electrochemistry with application in biosensing: A review. Biosens Bioelectron 2018;121:125–136. doi:10.1016/j.bios.2018.08.051
Sigolaeva LV, Bulko TV, Kozin MS, Zhang W, Köhler M, Romanenko I, et al. Long-term stable poly(ionic liquid)/MWCNTs inks enable enhanced surface modification for electrooxidative detection and quantification of dsDNA. Polymer. 2019;168:95–103. doi:10.1016/j.polymer.2019.02.005
Manna S, Sharma A, Satpati AK. Electrochemical methods in understanding the redox processes of drugs and biomolecules and their sensing. Curr Opin Electrochem. 2022;32:100886. doi:10.1016/j.coelec.2021.100886
Imanzadeh H, Sefid-Sefidehkhan Y, Afshary H, Afruz A, Amiri M. Nanomaterial-Based Electrochemical Sensors for Detection of Amino Acids. J Pharm Biomed Anal. 2023;230:115390. doi:10.1016/j.jpba.2023.115390
Zhang F, Gu S, Ding Y. Electrooxidation and determination of cefotaxime on Au nanoparticles. poly (L-arginine) modified carbon paste electrode. J Electroanal Chem. 2013;698:25–30. doi:10.1016/j.jelechem.2013.03.010
Compton B, Banks C. Understanding Voltammetry, 2nd ed.; Imperial College Press: London, UK, 2011. doi:10.1142/p726
Nicholson RS, Shain I. Theory of stationary electrode polarography. Single scan and cyclic methods applied to reversible, irreversible, and kinetic systems. Analyt Chem. 1964;36:706–723. doi:10.1021/ac60210a007
Bard AJ, Faulkner LR. Electrochemical Methods: Fundamentals and Applications; John Wiley & Sons Inc.: New York, NY, USA,2001.
Li R, Chen X, Zhou C, DaiQ-Q, Yang L. Recent advances in β-lactamase inhibitor chemotypes and inhibition modes. Eur J Med Chem. 2022;242:114677. doi:10.1016/j.ejmech.2022.114677
Robertson JG. Mechanistic Basis of Enzyme-Targeted Drugs. Biochem. 2005;44:5561-5571. doi:10.1021/bi050247e
DOI: https://doi.org/10.15826/chimtech.2024.11.4.07
Copyright (c) 2024 Victoria V. Shumyantseva, Tatiana V. Bulko, Galina V. Presnova, Vitaly G. Grigorenko, Maya Yu. Rubtsova

This work is licensed under a Creative Commons Attribution 4.0 International License.
© Website Chimica Techno Acta, 2014–2024
ISSN 2411-1414 (Online)
This journal is licensed under a Creative Commons Attribution 4.0 International