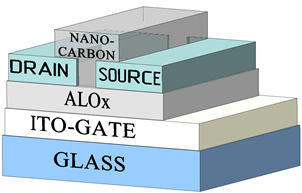
Nanofibrous carbon (multi-wall carbon nanotubes): synthesis and electrochemical studies by using field-effect transistor setup
Abstract
Synthesis of nanofibrous carbon (multi-wall carbon nanotubes, MWCNTs) by means of n-hexane pyrolysis is reported. The structure of the obtained MWCNTs was studied using scanning electron microscopy, and the diameters of 20–85 nm and lengths of 500–600 nm for these nanotubes were observed. By using the above mentioned MWCNTs field-effect transistors were fabricated on ITO glass substrates with a gate dielectric made of 390 nm thick Al2O3 foil and the drain-source contacts made of 300 nm thick aluminum foil. The Nano-C film 200 nm thick was deposited by thermal evaporation in vacuum. The properties of the obtained field-effect transistors were studied. The current-voltage characteristics of the OFET show an increase in currents with a positive voltage on the gate, which corresponds to the electron conductivity of the transport channel. The dependences are nonlinear, and there are no saturation regions in the output characteristics. The Raman spectra indicate the presence of nickel and show characteristic peaks for C=C and CH bonds.
Keywords
Full Text:
PDFReferences
Mishchenko SV, Tkachev AG. Carbon nanomaterials. Production, properties, application. Mashinostroenie. 2008; 320.
Mahato B, Lomov SV, Shiverskii A, Owais M. A review of electrospun nanofiber interleaves for interlaminar toughening of composite laminates. Polymers. 2023;15(6):1380 doi:10.3390/polym15061380
Lan G, Yang J, Ye RP, Boyjoo Y, Liang J, Liu X, Qian K. Sustainable carbon materials toward emerging applications. Small Methods. 2021;5(5):2001250. doi:10.1002/smtd.202001250
Egoro0v NV, Sheshin EF. Carbon-based field emitters: properties and applications. Modern Develop Vacuum Electron Sources. 2020;449–528.
Liu Y, Kumar S. Polymer/carbon nanotube nano composite fibers–a review. ACS Appl Mater Interfaces. 2014;6(9):6069–6087. doi:10.1021/am405136s
Kirmani MH, Jony B, Gupta K, Kondekar, Ramachandran J, Arias-Monje PJ, Kumar S. Using a carbon fiber sizing to tailor the interface-interphase of a carbon nanotube-polymer system. Composites Part B Engineering. 2020;247:110284. doi:10.1016/j.compositesb.2022.110284
Newcomb BA, Giannuzzi LA, Lyons KM, Gulgunje PV, Gupta K, Liu Y, Kumar S. High resolution transmission electron microscopy study on polyacrylonitrile/carbon nanotube based carbon fibers and the effect of structure development on the thermal and electrical conductivities. Carbon. 2023;93:502–514. doi:10.1016/j.carbon.2015.05.037
Karakashov B. Studies of fibrous carbons for high-temperature thermal energy storage (Doctoral dissertation, Université de Lorraine). 2019.
Kour R, Arya S, Young S-J, Gupta V, Bandhoria P, Khosla A. Review—Recent advances in carbon anomaterials as electrochemical biosensors. J Electrochem Soc. 2020;167:037555. doi:10.1149/1945-7111/ab6bc4
Nurmukhametova A, Zenitova L. Carbon fiber. Overview. Norwegian Journal of development of the International Science. 2022;86:64–96.
Mukhina TN, Barabanov NL, Babash ES et al. Pyrolysis of hydrocarbon raw materials, Chemistry. 1987; 240.
Popov MV, Golovakhin VV, Gudyma TS, Lazarenko NS, Lapekin NL, Shestakov AA, Bannov AG. A method for producing carbon nanomaterial and hydrogen (options) and a device for producing carbon nanomaterial and hydrogen in a continuous mode. Patent RU №. 2790169, 2023 (in Russian) [Popov MV, Golovakhin VV, Gudyma TS, Lazarenko NS, Lapekin NI, Shestakov AA, Bannov AG. Sposob poluche-niya uglerodnogo nanomateriala i vodoroda (varianty) i ustroystvo dlya polucheniya uglerodnogo nanoma-teriala i vodoroda v nepreryvnom rezhime. Patent RF №2790169, 2023].
Avdeeva LB, Goncharova OV, Kuvshinov GG et al. Method for producing hydrogen and carbon material applicant and patent holder: Institute of Catalysis named after. Patent RU № 2064889 (in Russian) 1996; [Avdeyeva LB, Goncharova OV, Kuvshinov GG i dr.; Sposob polucheniya vodoroda i uglerodnogo materiala: Patent RF № 2064889,1996.]
Ermakova MA, Ermakov DYu, Kuvshinov GG, Plyasova LM. New Nickel Catalysts for the Formation of Filamentous Carbon in the Reaction of Methane Decomposition. J Catal. 1999;187:77–84. doi:10.1006/jcat.1999.2562
Marina A, Ermakova, Dmitriy Yu, Ermakov, Chuvilin A. et al. Decomposition of methane over iron catalysts at the range of moderate temperatures: the influence of structure of the catalytic systems and the reaction conditions on the yield of carbon and morphology of carbon filaments. J Catalysis. 2001;201:183–197. doi:10.1006/jcat.2001.3243
Reshetenko TV, Avdeeva LB, Ismagilov ZR et al, Carbon capacious Ni-Cu-Al2O3 catalysts for high-temperature methane decomposition. Appl Catalysis A Gen. 2003;247:51–63. doi:10.1016/S0926-860X(03)00080-2
Kuvshinov GG, Zavarukhin SG, Mogilnykh YuL et al. Implementation of the process for producing granular catalytic fibrous carbon on a pilot reactor scale, Chemical Industry.1998;5:300–307 p. (in Russian) [Kuvshinov GG, Zavarukhin SG, Mogil'nykh YUL i dr., Realizatsiya protsessa polucheniya granulirovannogo kataliticheskogo voloknistogo ugleroda v masshtabe pilotnogo reaktora, Khimicheskaya promyshlennost'. 1998; № 5. s. 300-307].
Steinfeld A, Kirillov V, Kuvshinov G et al. Production of filamentous carbon and hydrogen by solarthermal catalytic cracking of methane. Chem Eng Sci. 1997:3599–3603. doi:10.1016/S0009-2509(97)00166-8
Kuvshinov GG, Parmon VN, Sadykov VA, Sobyanin VA. New catalysts and catalytic processes to produce hydrogen and syngas from natural gas and other light hydrocarbons. Studies Surface Sci Catalysis. 1998;19:677.
Yu T, Li J, Yao J, Kang L. Qingwen Li. Filled carbon-nanotube heterostructures: from synthesis to application. Microstructures. 2023; doi:10.20517/microstructures
Karousios N, Tagmatarchis N, Tasis D. Current Progress on the Chemical Modification of Carbon Nanotubes. Chem Rev. 2010;110:5366–5397.
MacKenzie KJ, Dunens OM, Harris AT. An Updated Review of Synthesis Parameters and Growth Mechanisms for Carbon Nanotubes in Fluidized Beds. Ind Eng Chem Res. 2019;49(11):5323–5338. doi:10.1021/ie9019787
See CH, Harris AT. A review of carbon nanotube synthesis via fluidized-bed chemical vapor deposition. Ind Eng Chem Res. 2007; 46(4):997–1012. doi:10.1021/ie060955b
Ermakova M, Ermakov D, Kuvshinov G et al. Decomposition of methane over iron catalysts at the range of moderate temperatures: the influence of structure of the catalytic systems and the reaction conditions on the yield of carbon and morphology of carbon filaments. 2001;201:183–197. doi:10.1006/jcat.2001.3243
Reshetenko T, Avdeeva L, Ismagilov Z et al. Catalytic filamentous carbons-supported Ni for low temperature methane decomposition. 2005;102:115–120. doi:10.1016/j.cattod.2005.02.011
Reshetenko TV, Avdeeva LV, Ushakov VA et al. Coprecipitated iron-containing catalysts (Fe-Al2O3, Fe-Co-Al2O3, Fe-Ni-Al2O3) for methane decomposition at moderate temperatures: Part II. Evolution of the catalysts in reaction. Appl Catalysis A Gen. 2004;270:87–99. doi:10.1016/j.apcata.2004.03.045
Reshetenko T, Avdeeva L, Ismagilov Z et al. Catalytic Filamentous Carbon: Structural and Textural Properties. Carbon. 2004;42:2501–2507. doi:10.1016/S0008-6223(03)00115-5
Takenaka S, Kobayashi HO. Ni/SiO2 catalyst effective for methan e decomposition into hydrogen and carbon nanofiber, J Catalysis. 2003;136:79–87. doi:10.1016/S0021-9517(02)00185-9
Ermakova M, Ermakov D. Ni/SiO2 and Fe/SiO2 catalysts for production of hydrogen and filamentous carbon via methane decomposition. 2002;77:225–233. doi:10.1016/S0920-5861(02)00248-1
Hazr M, Croiset E, Hudgins RR et al., Experimental investigation of the catalytic cracking of methane over a supported Ni catalyst. Can J Chem Eng. 2009;87:99–105. doi:10.1002/cjce.20087
Takenaka S, Shigeta Y, Tanabe E, Otsuka K. Methane decomposition into hydrogen and carbon nanofibers over supported Pd-Ni catalysts. J Catalysis. 2003;220:468–477. doi:10.1016/S0021-9517(03)00244-6
Mustafin IA, Galiakhmetov RN, Kurochkin AK, Akhmetov AF, Khanov AR. Termodestructive distillation of gas oil and fuel oil in the presence of ultrafine, metal-containing catalytic systems in pilot conditions. Chem Technol Fuels Oils. 2022;3:5–9. doi:10.32935/0023-1169-2022-631-3-5-9
Mustafin AG Galiakhmetov RN, Sudakova OM, Mustafin IA, Gimadieva AR. Method of producing nickel 2-ethylhexanoate. Patent holder: Bashkirskij gosudarstvennyj university. named after. Patent RU № 2612220 (in Russian) 2015; [Mustafin AG, Galiakhmetov RN, Sudakova OM, Mustafin IA, Gimadieva AR; Sposob polucheniya 2-ethylhexanoata nickelj: Patent RF № 2612220, 2015.]
Kamran U, Heo Y-J, Won Lee J, Park S-J. Functionalized Carbon Materials for Electronic Devices: A Review. Micromachines. 2019;10(4):234. doi:10.3390/mi10040234
Kim SH, Kang I, Kim YG, Hwang HR, Kim YH, Kwon SK, Jang J. High performance ink-jet printed diketopyrrolopyrrole-based copolymer thin-film transistors using a solution-processed aluminium oxide dielectric on a flexible substrate. J Mater Chem C. 2013;1(13):2408–2411. doi:10.1039/C3TC00718A
Avis C, Hwang HR, Jang J. Effect Of Channel Layer Thickness On The Performance Of Indium–Zinc–Tin Oxide Thin Film Transistors Manufactured By Inkjet Printing. ACS Appl Mater Interfaces. 2014;6(14):10941-10945. doi:10.1021/am501153w
Zhou Y, Wang X, Dodabalapur A. Dual-Band Perovskite Bulk Heterojunction Self-Powered Photodetector for Encrypted Communication and Imaging. Adv Electron Mater. 2022;2200786. doi:10.1002/adom.202200786
Tameev AR, Rakhmeev RG, Nikitenko VR, Salikhov RB, Bunakov AA, Lachinov AN, Vannikov A.V. Effect of excessive pressure on the drift mobility of charge carriers in poly(diphenylene phthalide) films. Phys Solid State. 2011;53:195–200. doi:10.1134/S106378341101032X
Zhou X, Wang Z, Song R, Zhang Y, Zhu L, Xue D. High performance gas sensors with dual response based on organic ambipolar transistors. J Mater Chem C. 2020;219(5):1584–1592. doi:10.1039/D0TC04843G
Kobayashi S, Takenobu T, Mori S, Fujiwara A, Iwasa Y. Sci Technol Adv Mat. 2003; 4. 371. doi:10.1016/S1468-6996(03)00064-0
Park B. Dielectric relaxation dependent memory elements in pentacene[6, 6]-phenyl-C61-butyric acid methyl ester bi-layer field effect transistors. Thin Solid Films. 2015; 578. 156–160. doi:10.1016/j.tsf.2015.02.043
Köhler А, Bässler Н. Electronic Processes in Organic Semiconductors: An Introduction. Wiley Weinheim. 2015.
DOI: https://doi.org/10.15826/chimtech.2024.11.4.21
Copyright (c) 2024 Ildar A. Mustafin, Arslan F. Akhmetov, Renat B. Salikhov, Ilnur A. Mullagaliev, Timur R. Salikhov, Rail N. Galiakhmetov, Olga V. Shabunina, Dmitry S. Kopchuk, gor S. Kovalev, Akhat G. Mustafin

This work is licensed under a Creative Commons Attribution 4.0 International License.
Chimica Techno Acta, 2014–2025
eISSN 2411-1414
Copyright Notice