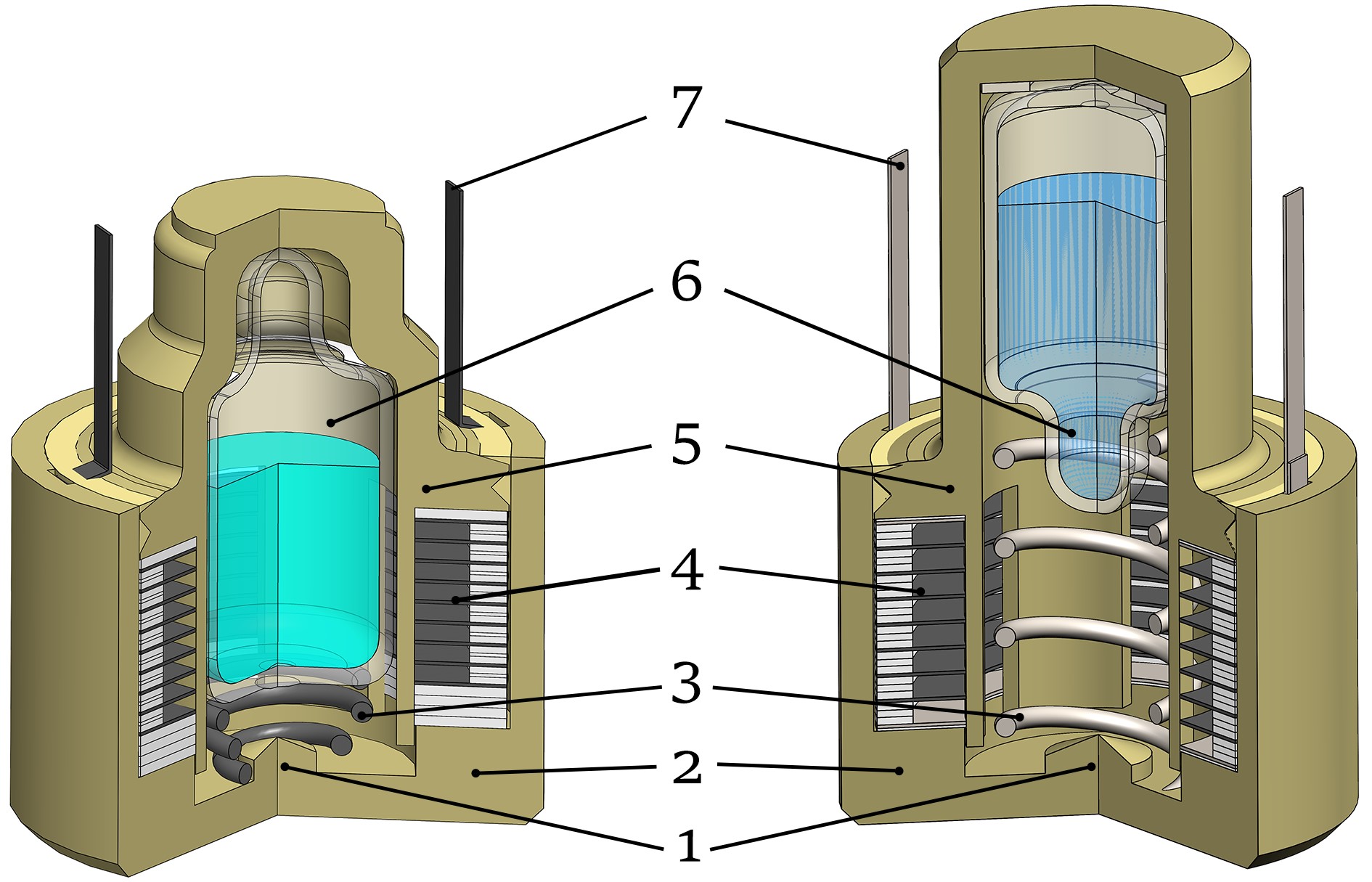
Fast-activating reserve power sources: is lead dead indeed?
Abstract
Keywords
Full Text:
PDFReferences
Beard KW, Reddy TB, editors. Linden’s Handbook of Batteries. 5th ed. New York, Chicago, etc.: McGraw Hill; 2019. 1456 p.
Bagotsky VS, Skundin AM, Volfkovich YuM. Electrochemical power sources: Batteries, Fuel Cells, and Supercapacitors. Hoboken NJ: John Wiley & Sons; 2015. 403 p. doi:10.1002/9781118942857
Ritchie AG. Military applications of reserve batteries. Philos Trans Royal Soc A. 1996;354(1712):1643–1652.
Clesca O. Tailoring the size and performance of a reserve lithium battery for the next generation fuzes. In: 56th National Defense Industrial Association Annual Fuze Conference ‘Next Generation Fuzing for Next Generation Weapons’; 2012 May 14–16; Baltimore MD, USA. 13708.
Straver J, inventor; Thales Nederland BV, assignee. Reserve batterij en de toepassingsmogelijkheden ervan. The Netherlands patent NL 1022034. 2004 Aug 02. Dutch.
Hein R. State of the art fuze batteries and their performance. In: 61th National Defense Industrial Association Annual Fuze Conference ‘Fuzing Solutions – A Global Perspective’; 2018 May 15–17; San Diego CA, USA. 20455.
Hein R, inventor. Diehl & Eagle Picher GmbH, assignee. Aktivierungseinrichtung für eine Batterie für einen elektronischen Zündmechanismus. Germany patent DE 102019004140. 2020 Dec 17. German.
Schisselbauer P, Wightman B. Lithium battery innovations for projectile munitions. In: 62th National Defense Industrial Association Annual Fuze Conference ‘Fuzing Innovations for Tommorrow’s Weapons’; 2019 May 13–15; Buffalo NY, USA. 21767.
Jeon KY, Yang IC, Park SH, Kim KH, inventors; Vitzrocell Co Ltd, assignee. Ampoule type reserve battery. South Korea patent KR 10-1293523. 2013 Aug 02. Korean.
Jeon KY, Yang IC, Kim KH, inventors; Vitzrocell Co Ltd, assignee. Reserve battery with improved prevention of insulation failure of positive terminal. South Korea patent KR 10-1818913. 2016 Sep 06. Korean.
Golembiovskii VS, Esiev RU, Kolpashchikov YuV, Pavlenkov AB, Chizhevskii OT, inventors; JSC ‘Scientific and Production Association ‘Pribor’, assignee. Energosoderzhashchii istochnik toka. The Russian Federation patent RU 2487313. 2012 Feb 03. Russian.
Shpekina VI. Development of the lead dioxide electrodeposition technology onto various substrates under ultrasonic field [dissertation]. Saratov (Russia): The Saratov state technical university; 2016. 136 p. Russian.
Brickwedde LH. Properties of aqueous solutions of perchloric acid. J Res Nat Bur Stand. 1949;42:309–329.
Yoon SH, Son JT, Oh JS. Miniaturized g- and spin-activated Pb/HBF4/PbO2 reserve batteries as power sources for electronic fuzes. J Power Sources. 2006;162(2):1421–1430.
Wich H. Lead is dead. In: 58th National Defense Industrial Association Annual Fuze Conference ‘Fuzing in a Challenging Environment’; 2015 Jul 7–9; Baltimore MD, USA. 17701.
Korotkov VA, Shpekina VI, Solovyova ND. The influence of ultrasound on nucleation in electrodeposition of lead dioxide on a nickel substrate. Electrochem Energetics. 2021;21(2):108–113. Russian. doi:10.18500/1608-4039-2021-21-2-108-113
Shpekina VI, Korotkov VA, Solovyova ND. Electrodeposition of lead dioxide on titanium substrate. Electrochem Energetics. 2021;21(4):191–196. Russian. doi:10.18500/1608-4039-2021-21-4-191-196
Shpekina VI, Korotkov VA, Solovyova ND. The effect of ultrasound on the coating properties and the kinetics of lead dioxide electrodeposition on the steel base. Electrochem Energetics. 2022;22(3):139–146. Russian. doi:10.18500/1608-4039-2022-22-3-139-146
Gorbachev NV, Gorbacheva EYu, Solovyova ND, Krasnov VV. The anodic behavior of electrodeposited lead and zinc in perchloric acid solution and the possibility of their use as anodes in reserve power sources. Electrochem Energetics. 2011;11(3):154–157. Russian. doi:10.18500/1608-4039-2011-11-3-154-157
Gorbachev NV. Technology of formation of anodic layers of electrodes of reserve power sources with perchloric acid [dissertation]. Saratov (Russia): The Saratov state technical university; 2011. 127 p. Russian.
GOST 9.305-84. Unified system of corrosion and ageing protection. Metal and non-metal inorganic coatings. Technological process operations for coating production [interstate standard]. Moscow (Russia): Izdatel’stvo standartov; 2003. 107 p. Russian.
GOST 9.302–88. Unified system of corrosion and ageing protection. Metal and non-metal in organic coatings. Control methods [interstate standard]. Moscow (Russia): Izdatel’stvo standartov; 2001. 38 p. Russian.
Volfkovich YuM, Bagotzky VS. The method of standard porosimetry: 1. Principles and possibilities. J Power Sources. 1994;48(3):327–338. doi:10.1016/0378-7753(94)80029-4
Volfkovich YuM, Bagotzky VS, Sosenkin VE, Blinov IA. The standard contact porosimetry. Colloids Surf A: Physicochem Eng Asp. 2001;187–188:349–365. doi:10.1016/S0927-7757(01)00650-1
Vol’fkovich YM, Sakars AV, Volinsky AA. Application of the standard porosimetry method for nanomaterials. Int J Nanotechnol. 2005;2(2):292–302. doi:10.1504/IJNT.2005.008066
Sukhotin AM, editor. Spravochnik po elektrokhimii [Handbook of Electrochemistry]. Leningrad: Khimiya; 1981. 488 p. Russian.
GOST R 58593–2019. Primary and secondary cells and batteries. Vocabulary [national standard of the Russian Federation]. Moscow (Russia): Standartinform; 2019. 66 p. Russian.
Prosyanyuk VV, Suvorov LS, Prudnikov NV. Direct chemical energy conversion of power-condensed systems into electric energy in combustion mode. Rossiiskiy Khimicheskiy Zhurnal. 2021;65(3):67–71. Russian. doi:10.6060/rcj.2021653.9
Prosyanyuk VV, Suvorov LS, Prudnikov NV, Gilbert SV, Zyuzina DS. On the possibility of expansion of the domestic component base of current sources based on energy condensed systems. Rossiiskiy Khimicheskiy Zhurnal. 2020;64(3):82–87. Russian. doi:10.6060/rcj.2020643.10
Vetter KJ. Elektrochemische Kinetik. Berlin, Heidelberg: Springer; 1961. 699 S. German. doi:10.1007/978-3-642-86547-3
Li X, Pletcher D, Walsh FC. Electrodeposited lead dioxide coatings. Chem Soc Rev. 2011;40(7):3879–3894. doi:10.1039/c0cs00213e
Kong J, Shi S, Kong L, Zhu X, Ni J. Preparation and characterization of PbO2 electrodes doped with different rare earth oxides. Electrochim Acta. 2007;53(4):2048–2054. doi:10.1016/j.electacta.2007.09.003
Velichenko AB, Amadelli R, Baranova EA, Girenko DV, Danilov FI. Electrodeposition of Co-doped lead dioxide and its physicochemical properties. J Electroanal Chem. 2002;527(1–2):56–64. doi:10.1016/S0022-0728(02)00828-8
Xu H, Yuan Q, Shao D, Yang H, Liang J, Feng J, Yan W. Fabrication and characterization of PbO2 electrode modified with [Fe(CN)6]3− and its application on electrochemical degradation of alkali lignin. J Hazard Mater. 2015;286:509–516. doi:10.1016/j.jhazmat.2014.12.065
Shcheglov PA, Samsonov DA, Pavlenkov AB, Kulova TL, Rychagov AYu, Nikolskaya NF, Shiryaev AA, Skundin AM. The effect of the positive electrode properties on the activation time of the lead–perchloric acid–lead dioxide-based reserve chemical power sources. Russ J Electrochem. 2023;59(12):1062–1070. doi:10.1134/S1023193523120121
Shcheglov PA, Samsonov DA, Pavlenkov AB, Kulova TL, Rychagov AYu, Andreev VN, Skundin AM. Investigation of physicochemical characteristics of lead dioxide coatings to enhance the performance of reserve quickly activated chemical power sources in the lead–perchloric acid–lead dioxide system. Russ J Phys Chem A. 2023;97(12):2835–2844. doi:10.1134/S0036024423120269
Pavlov D. Lead-Acid Batteries: Science and Technology: A Handbook of Lead-Acid Battery Technology and Its Influence on the Product. 2nd ed. Amsterdam: Elsevier; 2017. 720 p.
Pavlov D, Balkanov I, Halachev T, Rachev P. Hydration and amorphization of active mass PbO2 particles and their influence on the electrical properties of the lead-acid battery positive plate. J Electrochem Soc. 1989;136(11):3189–3197. doi:10.1149/1.2096424
Pavlov D. The lead-acid battery lead dioxide active mass: A gel–crystal system with proton and electron conductivity. J Electrochem Soc. 1992;139(11):3075–3079. doi:10.1149/1.2069034
Pavlov D. Influence of crystal and gel zones on the capacity of the lead dioxide active mass. J Power Sources. 1992;40(1–2):169–173. doi:10.1016/0378-7753(92)80049-H
Pavlov D, Petkova G, Dimitrov M, Shiomi M, Tsubota M. Influence of fast charge on the life cycle of positive lead-acid battery plates. J Power Sources. 2000;87(1–2):39–56. doi:10.1016/S0378-7753(99)00358-4
Dodson VH. The composition and performance of positive plate material in the lead-acid battery. J Electrochem Soc. 1961;108(5):406–412. doi:10.1149/1.2428101
Rüetschi P. Influence of crystal structure and interparticle contact on the capacity of PbO2 electrodes. J Electrochem Soc. 1992;139(5):1347–1351. doi:10.1149/1.2069410
Petersson I, Berghult B, Ahlberg E. Thin lead dioxide electrodes for high current density applications in semi-bipolar batteries. J Power Sources. 1998;74(1):68–76. doi:10.1016/S0378-7753(98)00035-4
Qu D. Fundamental principles of battery design: Porous electrodes. AIP Conf Proc. 2014;1597:14–25. doi:10.1063/1.4878477
Shcheglov PA, Samsonov DA, Pavlenkov AB, Sidorov YuM, Samoryadov AV. Application of innovative polymer composite materials based on polyphenylene sulfide in the design of power supply devices. Plasticheskie massy. 2023;(3–4):39–43. Russian. doi:10.35164/0554-2901-2023-3-4-39-43
DOI: https://doi.org/10.15826/chimtech.2024.11.1.03
Copyright (c) 2023 Pavel A. Shcheglov, Dmitry A. Samsonov, Anatoly B. Pavlenkov, Tatiana L. Kulova, Alexey Yu. Rychagov, Alexander M. Skundin, Evgeniya Yu. Postnova

This work is licensed under a Creative Commons Attribution 4.0 International License.
Chimica Techno Acta, 2014–2025
eISSN 2411-1414
Copyright Notice